Physiology and metabolism in the fossil record
But first, a detour: What are the biological implications of the snuggling dicynodonts?
Constraining the history of endothermy; Endothermy (warm-bloodedness) is considered a characteristic feature of mammals. What exactly is it and from how did it arise?
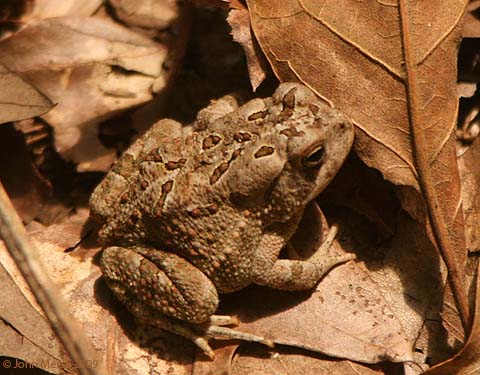
Fowler's toad - a bradymetabolic poikilothermic ectotherm
- Relies on external heat sources and sinks.
- Allows its body temperature to rise and fall with that of its environment.
- Is, at best, capable of only short episodes of intense activity.
Because of how these characteristics are distributed among living vertebrates, we think of tachymetabolic and homeothermic as going together, but that is an oversimplification. How is homeothermy achieved?
- Heat is a by-product of metabolism.
- Mitochondria in brown fat cells metabolize glucose and oxygen to generate heat.
- Muscle contractions, as mitochondria in muscle cells metabolize glucose
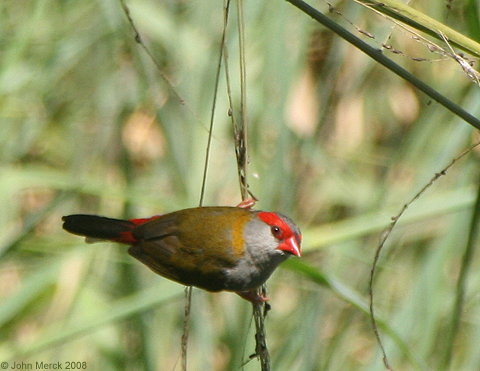
Red-browed finch - a tachymetabolic homeothermic endotherm
- Relies on internal heat sources
- Maintains a constant body temperature most of the time.
- Is generally capable of prolonged episodes of intense activity.
Thus, we are really talking about three separate capabilities:
- The ability to maintain constant temperature.
- The ability to exert large amounts of energy over a reasonably long time.
- The ability internally to generate body heat.
- Constancy of temperature:
- Homeothermic: Maintaining constant body temperature by any means.
- Poikilothermic: Allowing temperature to vary with environment.
- Source of heat:
- Ectothermic: Obtaining heat from environment.
- Endothermic: Generating heat internally.
- Activity levels:
- Tachymetabolic: With rapid high-energy metabolism.
- bradymetabolic: With slow, low energy metabolism.
Fundamentally, these abilities are determined by separate and distinct functions of the body, however there are some connections between them. As a result we are used to - bradymetabolic poikilothermic ectotherms (right above) and tachymetabolic homeothermic endotherm (right), but there do exist:
Energy pathways: There are two general ways in which a body cell can convert glucose into useful energy:
- Anaerobic respiration: Is relatively inefficient and yields toxic byproducts such as lactic acid, along with energy, but can proceed in the absence of oxygen.
- Aerobic respiration: Over three times as energy-efficient and without toxic byproducts, but requires significant amounts of oxygen.
Resting metabolic rate: The base rate at which an organism metabolizes glucose while at rest. In endothermic homeotherms like mammals, this can be roughly ten times what it is in ectothermic creatures.
Aerobic scope: The factor to which an organism can elevate its activity levels over the resting state without slipping into anaerobic respiration. For most mammals, that is 10 - 20. The aerobic champs are insects like hoverflies, some of which have aerobic scopes approaching 300.
How is this achieved. The body cells of animals are not that different in their ability to metabolize. Where animals differ is in the arrangement of their plumbing. For an animal to maintain a high metabolic rate, its cells must be well-supplied with:
- Oxygen
- Glucose
Glucose plumbing: To make more glucose available to its cells, an animal must:
- Digest efficiently by reducing food to small bites before digestion or employing chewing or gizzards to grind food up.
- Eating more food: Once digestion is efficient enough to yield more energy, one can hunt more effectively and capture more food.
- Moving more air through its lungs by increasing the efficiency of ventilation. In mammals, ventilation is aided by a muscular diaphragm and the separation of food and air in the mouth.
- Separating oxygenated and deoxygenated blood: by means of a four-chambered heart.
The connection to temperature: Metabolic reactions are mediated by protein catalysts that are sensitive to temperature:
- Too cold and the catalysts will work less effectively, slowing down metabolism.
- Too hot and the catalysts will break down, causing metabolism to cease.
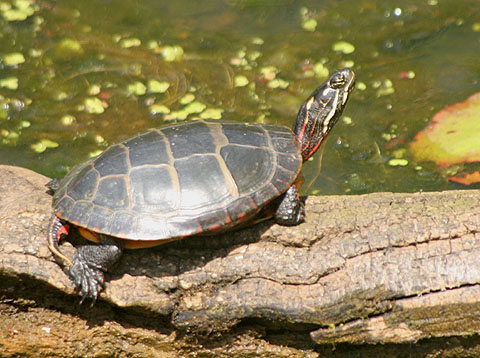
Eastern painted turtle soaking up the sun
- The environment: Find a warm spot and lie in it. Especially useful for small animals with high surface area/volume ratios, able to exploit microenvironments. Many amniotes begin their days by basking in the sun capture enough heat to achieve peak metabolic efficiency.
- Metabolic heat: If your resting metabolic rate is high enough, you warm yourself by burning glucose. Big connection: animals with glucose "to burn" can choose when to become active regardless of external temperature, thereby increasing their ability to capture more glucose.
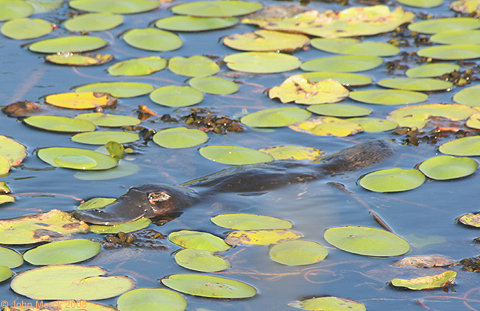
Duck-billed platypus cooling off
- Environmental heat sinks: Find a cool spot and lie in it. Especially useful for small animals with high surface area/volume ratios, able to exploit microenvironments. Many amniotes retreat to shade or bodies of water to avoid overheating. Larger animals may alter their surface to volume ratio by growing "cooling fins" to facilitate environmental heat exchange.
Where have you seen this?
Nasal turbinates of musk ox - Evaporative cooling: Increase the thermal conductance of your surface by moistening it. Can take the form of:
- Sweat
- Respiratory surfaces: The combination of panting - moving large volumes of air across the moist linings of the oral cavity, pharynx, etc, facilitates evaporative cooling, especially when combined with specialized evaporative cooling structures like nasal turbinates - scroll-shaped bones in the nasal cavities of mammals. (right)
Would this approach have been more convenient than using a Dimetrodon-like cooling fin?
Would this approach to cooling have had any effect on other aspects of metabolism that we have discussed?
Thus, adaptations for maintaining constant body temperature and adaptations for rapid metabolism tend to be mutually reinforcing.
Animals that have mastered that challenge of dumping excess heat may evolve integumentary systems like fur or feathers to limit the loss of metabolic heat through the skin.
Somewhere between the first synapsid and the first mammal, the transition from ectothermy to endothermy occurred. Some speculation:
- Dry skin: Frogs and salamanders have little ability to elevate their body temperatures above ambient levels, even by ectothermic means, because of their need to keep their skin moist to facilitate cutaneous breathing. This subjects them to evaporative cooling any time they come into open air. In contrast, even "cold blooded" amniotes can maintain an elevated body temperature while active because their skin is dry and impermeable. Achieving this was the first step in terrestrial thermoregulation. When did it occur? Once indication: ectothermic herbivores must maintain high body temperatures to facilitate digestion. The presence of large herbivorous diadectomorphs and basal synapsids suggests that the last common ancestor of diadectomorphs and amniotes had progressed that far.
- Cooling fins: Larger animals with lower surface area/volume ratios heat up and cool down too slowly. To accelerate morning activity and remain active at midday, heat exchange surfaces evolved, including the "sails" of sphenacodontines like Dimetrodon. (Of course, this structure could be used for display purposes, also.) But note: The presence of the sail indicates that Dimetrodon lacked the means to either heat or cool itself internally.
- Posture: For Dimetrodon, there was little difference between standing tall and sprawling flat. They didn't have to invest much energy in "standing up" before taking a step. Therapsids were different. With relatively short torsos, longer legs, and postures that brought the feet close to being underneath the body when they stood up, These were creatures that had to make a significant investment of energy in "standing up" before walking. One expects that, having made that investment, they would engage in longer bouts of walking around than their basal synapsid ancestors. That requires the energy we associate with tachymetabolism. How elevated was their body temperature when they were active or at rest? Good question.
- Ventilation: When did the muscular diaphragm appear? One indication is the separation of the torso into distinct thoracic and abdominal regions. We begin to see the differentiation of dorsal vertebrae and ribs reflecting this at the base of Cynodontia. What about evaporative cooling mechanisms? Only mammals have fully ossified nasal turbinates, however non-mammalian cynodonts and a few of their close relatives have ridges in their nasal cavities that seem to mark the attachment points for cartilagenous turbinates.
- Fur: Alas, we depend on rare fossil occurrences. We know this much:
- Sail-back sphenacodontines would absolutely not have had it.
- Cynodonts similar to Morganucodon very close to the ancestry of mammals definitely had proper fur. (E.G.: Castorocauda fossil and reconstruction.)
The overall picture is that non-therapsid synapsids were ectothermic in the style of lizards. A general increase in metabolic energy seems to have been achieved among therapsids, enabling more prolonged activity. Outward signs of ectothermic heat exchange with the environment are absent. Indications of advanced respiratory features are associated with cynodonts. Mammalian-style endothermy had been achieved before the origin of proper Mammalia.