The Reality of Climate Change Dawns
Climate change as a reality of nature: The first reliable thermometers went into use in Italy in the late 17th century. The first continuous records of daily temperature didn't begin until the early 19th century in England, so how do we know about ancient temperatures? In fact, by the 18th century, literate people recognized that climate conditions described by Classical and Medieval authors were often different from those that they witnessed. Today we note that the canals of the low countries are no longer the reliable winter highways for skaters depicted by Pieter Brueghel or described in Hans Brinker, or the Silver Skates. It is, therefore, apparent that climate changes over time. As the science of geology arose, the attention of geologists was drawn to ancient climates for which no eye-witness accounts existed.
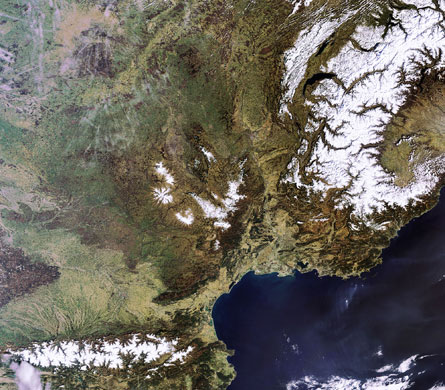
Physical Evidence for Glaciers
To know what kind of landforms led to this conclusion, one needs to understand the deposition of continental glaciers today:
Land forms resulting from continental glaciers: Continental scale glaciation creates interesting opportunities for ice to interact with large volumes of sediment. Note: Glacial sediment takes three forms:
- Till: unsorted glacial sediment deposited directly by ice.
- Stratified drift: Glacial sediment that has been subjected to some degree of sorting by outwash streams. Note: Glaciers don't sort sediment by size or round their corners at all. All streams of water, however, do to some extent.
- Erratics: Large boulders sitting out where they have no business.
Results include:
- Drumlin: Hills made of reshaped glacial till (unsorted glacial sediment deposited directly by ice).
- The steep end is on the side of the ice's approach
- The shallow end points in the direction of ice movement
- Kame [Scots"comb." Pronounced like English "came"]: Hills of stratified drift that form when a stream deposits sediment in a hole in the glacial ice.
- Kettle lake: This is essentially the opposite of a kame. When a block of glacial ice is stranded by a retreating glacier, it prevents sediments from being deposited. When if finally melts, a depression is left that fills with water. Think of Minnesota - the land of 1000 lakes. these lakes are mostly kettles formed during the retreat of a continental ice sheet.
- Esker [Irish Eiscir - "ridge"]: Long sinuous ridge of stratified drift. Results from sediment deposited in the point bars of under-glacier stream.
- Moraines Piles of glacial till transported to the edge of the glacier by flowing ice, then deposited as that ice melts.

Periglacial features
Beyond features created by glacial ice, itself, the regions adjacent to continental glaciers display characteristic features owing to:
- Permafrost in subsurface - that prevents water from infiltrating into soil.
- Intense freeze-thaw cycles at surface - That cause that water to modify soil landforms
Resulting land-forms reflect interaction of soil and ice:
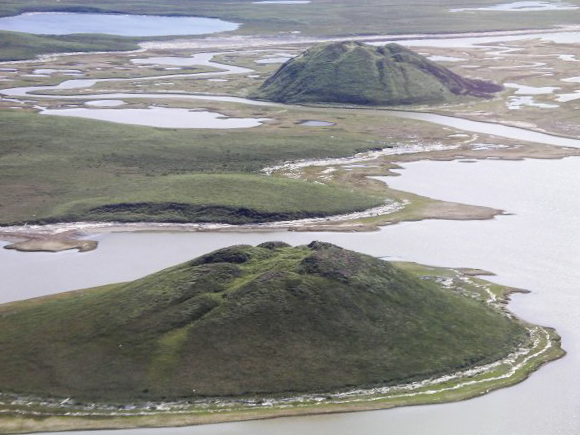
Proxy Data for ancient temperature
The problem with the glacial landforms discussed above is that they are very blunt instruments:
- They don't record temperature precisely
- New glaciers bulldoze away the traces of older ones.
- The oxygen and carbon stable isotope record
- The use of the distributions of climate-sensitive fossil organisms
- Bore-hole temperature data
- etc.
The Oxygen isotope record: During the late 20th century, a new technique allowed us to refine this sequence. The method:
- There are three stable (i.e. non-radioactive) isotopes of oxygen. The most important are 16O and 18O.
- When ocean water evaporates, molecules containing the lighter isotope of oxygen, 16O, are more likely to "take off" and enter the vapor phase.
- Normally, this wouldn't cause any permanent change in the oceans' isotopic chemistry because the molecules would soon return to the ocean as rain. During an ice age, however, the water is locked up in glacial ice, so the oceans become isotopically heavy.
- Now the magic: Microscopic critters are constantly sampling the oceans' oxygen by building it into their CaCO3 shells. When they die and fall to the bottom, they create a record of oceanic oxygen isotopes during their lives.
- We can reconstruct the ocean's isotopic history by looking at the ratio of oxygen isotopes present in their shells deposited at different times. That ratio, in turn, tells us how much water was locked up as continental ice.
Ice ages:
Putting this together reveals that climate over the last two million years has alternated between glacial and interglacial states, with atmospheric CO2 (plotted over the last 0.8 my, right) serving as a good general proxy for temperature.
We note the following:
- Glacials: Intervals of intense and extensive continental glaciations, characterized by dramatic climate fluctuations and low average temperature.
- Interglacials: Interval of relatively constant warm climate with less continental glaciation. We have been in the Holocene interglacial for the last 11,700 years.
Ice-ages: Longer interval characterized by the prolonged alternation of glacials and interglacials. Earth has been in an ice-age for the last 2.5 million years, roughly.
Note: The end of each glacial interval is marked by an abrupt warming. As the precision of our measurements improves, we find this transition to be more and more abrupt - measurable on the order of decades. Transitions to glacial conditions are less abrupt.
Condition 18,000 years ago during the last glacial maximum:
- Beringia - the land bridge across the Bering straits
- Australia, Tasmania, and New Guinea were one large land mass
- Many of the islands of Southeast Asia were part of the mainland.
How does climate shift between glacials and interglacials?
To address this we need to consider three factors:- Solar forcings: Changes in the amount of insolation that ultimately power climate change.
- The effects of continental positions: That determine whether there is any possibility for the formation of continental glaciers
- Feedback effects: That amplify the effects of solar forcings.
Solar forcings:
Milankovitch cycles: In the 1920s, the Yugoslavian meteorologist Milutin Milankovitch realized The Earth's movement through space is subject to three kinds of cycles:
- Orbital eccentricity: The orbit around the Sun is an ellipse that changes shape (becoming more and less circular) in a cycle of 100,000 years.
So, if northern hemisphere summer fell at the point in Earth's orbit when Earth is farthest from the Sun, summers would be milder at times of greater orbital eccentricity.
- Axial inclination: The axis of rotation is tilted. The angle of tilt varies from 21.5 deg. to 24.5 deg. in a cycle of 41,000.
So, the summer hemisphere would get more solar energy when axial inclination was greatest.
- Axial precession: The axis of rotation wobbles around an axis like that of a toy top. So, today the axis points toward Polaris, the north star, but 14,000 years ago, Vega was the north star. One full precessional wobble takes 23,000 years.
So, at a given point in Earth's orbit, the hemisphere experiencing summer today will be the winter hemisphere in 11,500 years.
Note: These parameters effect different latitudes differently at any given time.
Solar forcing: The sum of the effects of these cycles at a given latitude gives the general tendency for glaciers to form. Solar forcings are different at different latitudes and in different hemispheres. The graphic at right shows cumulative solar forcings for 65 deg. north, where most of Earth's land is concentrated.
Formation of continental glaciers:
Solar forcings are irrelevant unless they favor mild summers in high latitudes: Each cycle influences the severity of winters to some degree, but what really matters is the mildness of summers. It snows hard in many places, and in polar regions, it always snows during winter. That's irrelevant. Glaciers only form in regions where at least some of the previous winter's snow survives the summer. Thus, when Milankovitch cycles favor mild summers, as when:- orbital eccentricity is great,
- axial inclination is reduced,
- axial precession is such that summer comes when Earth is farthest from the sun,
World Geography, Greenhouses, and Ice-houses:
Ice-house world conditions prevail: Over deep time, Earth's pattern of ocean circulation has alternated between:
- Ice-house-world: Where ocean water circulation is driven by refrigeration and the formation of deep ocean water near the poles. Today's oceans, circulated by the formation of North Atlantic Deep Water (NADW) and Antarctic Bottom Water (ABW) is an ice-house world. (Do not confuse with "ice ages.")
- Greenhouse-world: Where ocean water circulation is driven by the density of warm hypersaline waters that form near the equator and spread northward. Dr. Holtz's precious Cretaceous was a greenhouse-world in which:
- Global currents could circle the world again and again without having to come near the poles
- The northward spread of warm equatorial bottom water spread equible climates into polar regions.
- There was neither continental ice, nor polar winter sea ice. It was an ice free world except for high mountain peaks.
The slight differences in summer weather caused by Milankovitch cycles doesn't make a lick of difference in greenhouse world conditions in which no winter snow has a prayer of surviving the summer. Thus, ocean circulation must bring water to the poles before we can think about having glaciations. Moreover....
Large land masses (snow-catchers) must exist in high latitudes Consider past ice ages:
- Quaternary Period:, The northern continents are close to the North Pole and Antarctica is at the South Pole.
- Carboniferous Period: The southern half of Pangea lay over the South Pole.
The process and its feedback effects:
If the above conditions are met, then the interaction of the Milankovitch cycles can initiate a glaciation. That is only the beginning. The factors that cause glacials form a positive feedback loop. In the following schematics, keep track of these variables:
- Incoming solar radiation: Controlled by Milankovitch cycles or (possibly) changes in the sun's actual output.
- Albedo: The Earth's reflectivity. Ice's albedo is very high. That of ocean water is low. The more sunlight gets reflected into space, the cooler the climate.
- Greenhouse gas concentration: Especially CO2. These are consistently erupted from volcanoes, regardless of the climate.
- CO2 weathering reactions: Atmospheric CO2 (in a series of steps) interacts with rocks in weathering reactions, yielding CaCO3. The CaCO3 typically ends up in the oceans, where it can be taken up by critters or precipitated as chemical sediments. Either way, atmospheric carbon is moved into the solid Earth.
A Glacial in a Nutshell: We keep it simple, without considering the effects of the biosphere.




The end? Only until solar forcing upsets the equilibrium again.
How long does it take? From the time that the ice starts to melt and CO2 weathering reactions can resume, it takes up to 150,000 years for the atmosphere to return to equilibrium. Why doesn't the CO2 just dissolve in the oceans? Much of it does, but to get it into the deep oceans requires passing it through the small oceanic "windows" where surface waters sink into the depths. (Today = NADW and ABW.) That also takes millenia.
The Warming phase:
The recoveries at the end of glacial intervals tend to happen rapidly. Just as cooling triggers a positive feedback loop over a short time scale, so does warming:
- Incoming sunlight warms summer hemisphere causing ice to begin to melt.
- Warming oceans can hold less CO2, so this comes out of solution, increasing atmospheric greenhouse effect.
- On warming continents, decomposition of (previously frozen) plant material releases more CO2.
We see that just as the albedo of growing ice sheets amplified cooling through solar forcing, the release of CO2 from oceans and biosphere amplifies warming through solar forcing.
Fine-tuning cycles within cycles:
Heinrich events: Within the ~100,000 year glacial-interglacial cycle hide smaller, more subtle cycles. Sedimentologists note that during the Quaternary, we see occasional deep ocean sediments full of glacial dropstones, a sign that during an interval of less than 1000 years, vast flotillas of icebergs detached from glaciers and spread out to melt in the northern oceans. Heinrich events occur on average every 7000 years. Cause is debated, but may be connected with complex chains of events where:
- Warm northern hemisphere currents are deflected southward by the injection of fresh meltwater causing:
- northern oceans to cool and adjacent glaciers to expand...
- causing the southern oceans to warm...
- Warmer southern oceans cause antarctic ice to melt...
- raising sea-level globally
- lifting the edges of northern glaciers off their bedrock, causing profusion of icebergs.
A final note: The climate changes associated with glacials and interglacials occur on an ecological time-scale, allowing critters to respond by changing their geographic ranges. Something about the beginning of the Holocene, however, was different and resulted in an (almost) world-wide mass extinction of large mammals. We will discuss this next week.