The Biomechanics of Flight
John Merck
General Aerodynamics
The airfoil:
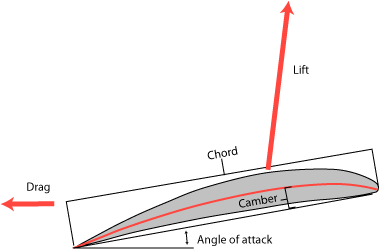
- Lift: The upward acceleration caused by the pressure differential above and below the airfoil. The faster the air flows over the wing, the greater the lift.
- Drag: The dissipation of locomotor energy through physical interactions with the fluid through which it moves. This comes in three forms:
- Frictional Drag: Locomotor energy dissipated by the friction of the fluid medium against the surface of the flying machine (animal or mechanical). Any animal that moves through a viscous fluid medium has to deal with the effects of frictional drag expressed by the Reynolds Number - a function of viscosity and speed. Air is significantly less viscous than water, however, fliers operate at such high speeds that frictional drag is often a significant constraint for them.
- Form drag: Drag resulting from the size of the profile of the flyer in anterior view. (A blimp would experience more of this than a rocket of the same length.)
- Induced drag: A special problem for flyers. Flyers employing an airfoil also experience drag caused by the formation of vortices at wingtips, as air rushes from below into the low-pressure region above the wing. As a result, the lifting effect of the wingtips is lost and the flier must drag the volume of air entrained in the vortex along with it. For flying animals and aircraft, the energy used to form these vortices is a significant energy sink. Aeronautical engineers have, in recent decades, employed wingtip winglets as "fences" to prevent vortex formation. (Compare modern Boeing 737 with one from the 1970s.)
- Frictional Drag: Locomotor energy dissipated by the friction of the fluid medium against the surface of the flying machine (animal or mechanical). Any animal that moves through a viscous fluid medium has to deal with the effects of frictional drag expressed by the Reynolds Number - a function of viscosity and speed. Air is significantly less viscous than water, however, fliers operate at such high speeds that frictional drag is often a significant constraint for them.
Modifying lift:
In any flying machine, lift can be increased by increasing:- Camber: the mean "height" of the airfoil perpendicular to its length. But this increases form drag.
- Angle of attack: the angle between the orientation of the airfoil and its direction of motion. But this increases form drag and eventually results in stalling - loss of fit due to the separation of air flow from the wing's upper surface.
- Wing area: For flyers of the same weight, greater wing area results in diminished wing loading, the ratio of weight/wing area. This reduction is beneficial because the wings must support the mechanical load of the entire flying machine, however it creates structural complications.
Problems with increasing lift:
- Induced drag: Any strategy that increases lift increases induced drag. Thus, flight involves tradeoffs between lift and drag.
- Stalling: Under most circumstances, lift only happens when air flows smoothly across the wing in laminar flow. If the angle of attack becomes too great, the air flow separates from the airfoil, resulting in the turbulent backflow of entrained air forward onto the upper surface. When this happens, lift disappears. The stall speed, the minimum speed at which an object can stay airborn, is inversely proportional to the amount of lift it generates. Stated differently: the less lift an animal's wings generate for its mass, the faster it must fly to stay aloft.
Modifying drag:
In any flying machine, drag can be diminished by:- Reducing camber
- Reducing angle of attack
- Reducing wing area

- increasing wing span: For a given wing area, a greater wing span, especially if wingtips are tapering, results in smaller vortices and reduced induced drag. (This more than compensates for increase in form drag.) Of course, for a given wing area and loading, increasing the wing span results in a wing with a higher aspect ratio (recall from our discussion of thunniform caudal fins.)
Problems with aspect ratio:
If we can reduce drag by increasing wing span without sacrificing wing area or increasing wing loading, why not just go for it and give every flyer wings like a high-performance sailplane? Alas, this, too, involves tradeoffs:

- The wing as a lever: The wing is subject to mechanical forces, especially shear. Indeed, the force of lift acts to shear the wing off of the body. As the wingspan increases, so does that shearing force's leverage. Eventually, a critical point is reached at which the wing skeleton (be it bone or titanium alloy) fails and the wing breaks.
- Turning loads: So far we have only considered the simple forces of lift and gravity on the wing:
- If these are equal, the machine flies in a level path,
- if lift exceeds gravity, the machine climbs,
- if gravity exceeds lift, the machine sinks.
Synopsis of the major trade-offs:
- Increased lift vs. stalling risk
- Lift vs. (vortex-) induced drag.
- Reducing drag by increased wingspan vs. increased shearing loads.
Propulsion:
Parachuting and gliding: Creatures like gliding frogs, snakes, squirrels, etc. store potential energy as they climb to their launch sites, then exchange it for the kinetic energy for propulsion. Once they take off, they can use only the stored energy of gravity.
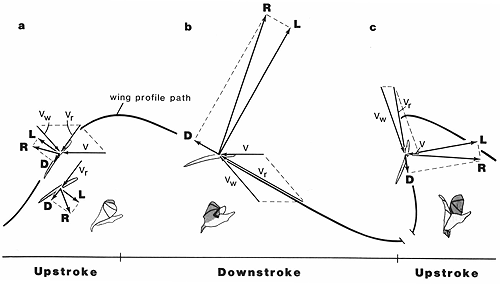
Powered flight is different. In terms of energy required per unit time, powered flight is the most expensive mode of locomotion. Why bother? Because it is fabulously efficient means of transport when measured as units of mass transported over units of distance.
Requirements: Fundamentally, unlike in gliders, the wing must provide both lift and propulsion.
This means that:
- The wing must be able to move so as to provide forward thrust. (Typically done as in subaqueous flyers, through the induction of lift on the forward surface as the wing is flapped.) In all powered flyers, the wingtips function as propellers, rotating so that lift will be concentrated on the leading surface. In birds, individual flight feathers are asymmetrical and rotate similarly. Usually, the distal portions of the wing are specialized for propulsion.
- It must be structured so that its shape and position can be finely controlled.
- Structural support must be concentrated at the leading edge of the wing, where bending loads are the most severe.
- Wings must be long enough to avoid excessive induced drag
- Their structure must be very light but capable of withstanding significant bending forces
- They must be moved by a powerful muscular system
- the creature's weight must be minimized.
Diversity of powered fliers:
In the history of animals, only four groups have effectively slipped the surly bonds of Earth:- Pterygote (winged) insects: (Starting ~325 mya) Employ two pairs of wings made of two stiff, flexible cuticle layers with blood vessels sandwiched in between, serving as structural elements.
- Pterosaurs: (Starting ~225 mya) Forelimbs support a patagium of varying proportions by means of an enlarged fourth finger. The unique pteroid bone supports the leading edge in front of the forearm. The patagium is invested with parallel collagen fibers, giving it the mechanical characteristics of a folding fan.
Pterosaurs' limb skeletons were hollow and invaded by pneumatic cavities. Their flight muscles arose from large sternums similar to those of birds.
- Birds: (Starting ~150 mya) Forelimbs support a wing formed of overlapping contour feathers. The outer wing consists of primary flight feathers supported by a hand with three coosified fingers.
Modern birds' limb skeletons are hollow and invaded by pneumatic cavities. Their flight muscles originate on large sternums. Uniquely, both the depressors and elevators of the wing arise from this region.
- Bats: (Starting ~50 mya) Fore and hind limbs support a flexible membranous patagium. The outer wing is supported by elongate digits II through V.
Whereas birds' and pterosaurs' limb skeletons are hollow, those of bats are solid but extremely slender. Among bats, the wing depressors originate on the sternum while the elevators arise from the scapulae.
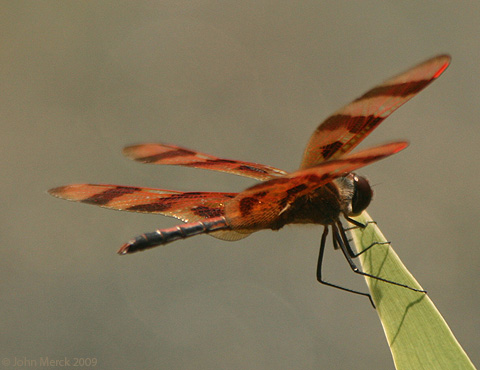
Lift enhancement: Depending on the construction of the wing, powered flyers are able to enhance life by several methods:
- Variable camber: The camber of membrane wings is easily altered.
- Bats can depress their thumb and digit II to lower the leading edge, while curling fingers IV and V to increase curvature of the trailing edge.
- Pterosaurs could depress their pteroid bone and digits I - III to lower the leading edge. Fossils indicate that the membrane was invested with muscles, giving the trailing edge some flexibility.
- Leading edge flaps:
Large aircraft operating near stall speeds often extend leading edge flaps that create a slot through which air is directed across the wing surface more precisely. Birds have an analogous structure, the alula. This is the homolog to the thumb, and supports a tuft of feathers that can be elevated to create a leading edge slot. Some insects achieve a slotted configuration through the close apposition of fore and hindwings (E.G. orthopterans).
Reduction of induced drag: Depending on trade-offs with other demands of flight, fliers use a range of strategies.
- Flyers who don't typically experience high turning loads can affort to have long tapering wings with narrow tips.
- In birds who must turn tightly in flight, primary feathers project out individually from the wingtips, the formation of wingtip vortices is suppressed as each feather acts as a separate small, lift-generating wing, each with its own tiny vortices.
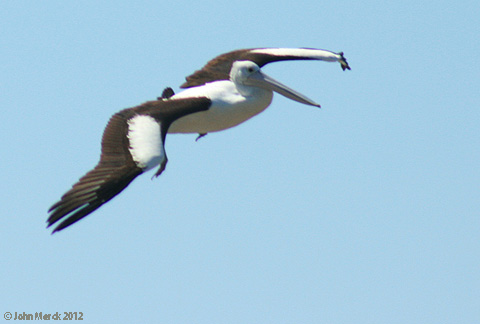
Scaling issues:
It goes without saying that in isometrically scaling fliers, wing area (and, therefore, lift) scales up as a square function while mass scales as a cube. Thus, we typically see wings and wing muscles scaling with positive allometry in nature. The critical point is reached when the body can't physically support the wings or the shearing loads that they must bear.Selective pressure to develop maximum flight thrust is such that in most birds, the major flight muscles already occupy a maximum proportion of overall body mass, regardless of size. Thus, they cannot be scaled up allometricallys. This places a limit on the overall size of an exclusively powered flyer of roughly 12 kg. - roughly the size of the largest powered flyers such as:
Anything bigger must cheat.
Adaptations to specialized flight strategies:
Slow flight and maneuverability:
Typical of birds and bats that maneuver through branches and other obstacles and have no pressing need for speed (passerines and vespertilionids). Characteristics:
- Large but low aspect ratio
- low-load wing (buoyant flight)
- large alula and slotted tips in birds
- high camber in bats.

High-speed flight:
Typical of:
- Creatures that are specialized to pursue prey at high speed:
- optimize their wings to generate thrust
- do not need to optimize lift because they are moving fast anyway.
- Birds that, for ecological reasons possibly unrelated to flight, have proportionally heavy bodies and are, therefore, constrained to relatively small wing areas simply must cope with high wing-loading and must fly fast to stay airborne. Includes:
- High aspect ratio
- high-load wing
- Unslotted tips in birds
- Low camber in bats.
Soaring: Many larger bodied birds get around the size limitations of the scaling of muscle mass by exploiting rising air masses in which they can glide (with a glide angle greater than zero) while still rising relative to the ground. To soar in a straight path, the optimum form is a low-load high aspect ratio wing, however many soarers are not like this. This disparity is because there are three distinct ways to soar:
- Thermal soaring:
Over warm land, rising columns of air form, with the fastest currents toward the center. Birds that ride thermals must be able to turn tightly in order to stay as near the center of the thermal as possible. Thus, their wings must bear heavy shearing loads. In order to avoid the shearing loads that would go with a high aspect ratio wing, they typically opt for shorter wings with slotted tips. (E.G.: turkey vulture) Thus they cope with many small vortices rather than one big one.
- Slope soaring:
When the wind encounters a large obstacle (E.G.: a cliff, ship, or large wave) a standing updraft is formed on its windward side. If the obstacle is elongate (like a mountian ridge or a large ocean wave), slope soarers can ride it considerable distances in more or less straight lines. This reduces shearing loads. Many terrestrial thermal soarers also slope soar, but slope soaring specialists are usually sea birds with long, high aspect ratio, high-load wings. (E.G.: waved albatross)
- Dynamic soaring: Dynamic soarers exploit the fact that wind speeds diminish closer to the ground. This happens in a series of steps:
- A bird approaching the ground while gliding downwind at a constant ground speed experiences increasing airspeed and lift - because the air around it is slowing down relative to the ground while the bird is not.
- Using this increased lift, the bird rises and turns into the wind. Again, the air speed increases because, as the bird rises, facing upwind, it encounters stronger winds.
- When it has reached a height at which the winds are effectively constant, and its drag has slowed it down, reducing its lift, it turns downwind and starts over.

Discussion:
Item 1: Confusciusornis sanctus
Item 2: Rhamphorhynchus muensteri
Item 3: Archaeopteryx lithographica
Item 4: Pteranodon longiceps